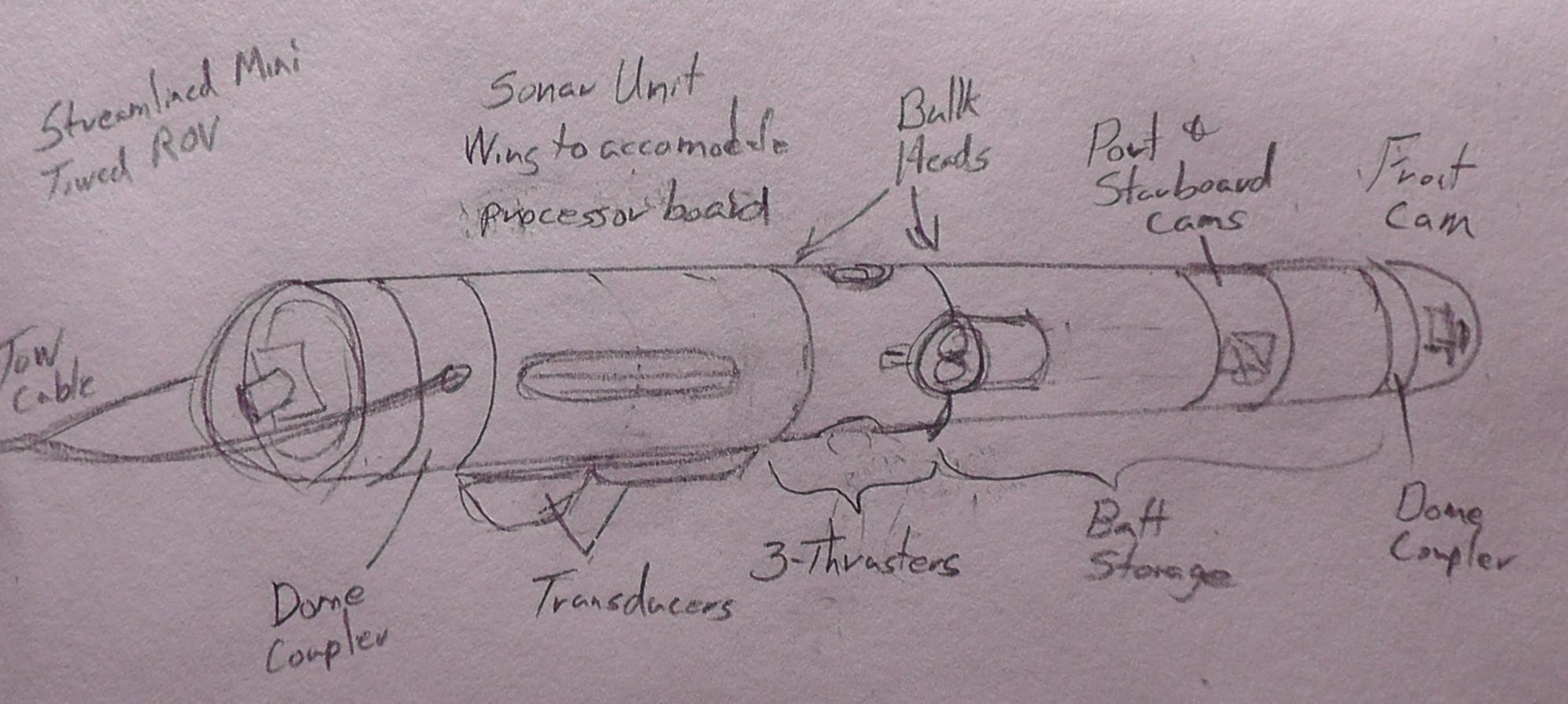
Hull Design 1
A small diameter hull can better resist crushing and has a smaller cross-section that reduces drag, but too small of a diameter and there is not enough displacement for the hull to be naturally buoyant. We selected 3 1/2″, Sch 40, 6061 T6 aluminum pipe providing 2.16 lbs of positive buoyancy per foot and a theoretical crush depth of 6,919 feet. The 4″ outside diameter of 101.6mm can accommodate the thrusters, and the inside diameter of 3 1/2″, 88mm will accommodate a VDSL2 transceiver, Ethernet hub, and cameras. Additional buoyancy or equipment can be added if needed by lengthening the hull. The hull is divided into seven compartments housing cameras, thrusters, lights, batteries and electronics. The three sections with thrusters and lights are free flooding so seven sections alternate between dry and wet. Couplers join the sections and incorporate bulk head connectors for wiring. Syntactic foam made from epoxy and glass spheres and/or a a lead keel internal to the hull would provide resistance to roll. There are two battery compartments, one forward and one aft, so pitch can be adjusted by moving batteries from one compartment to the other.
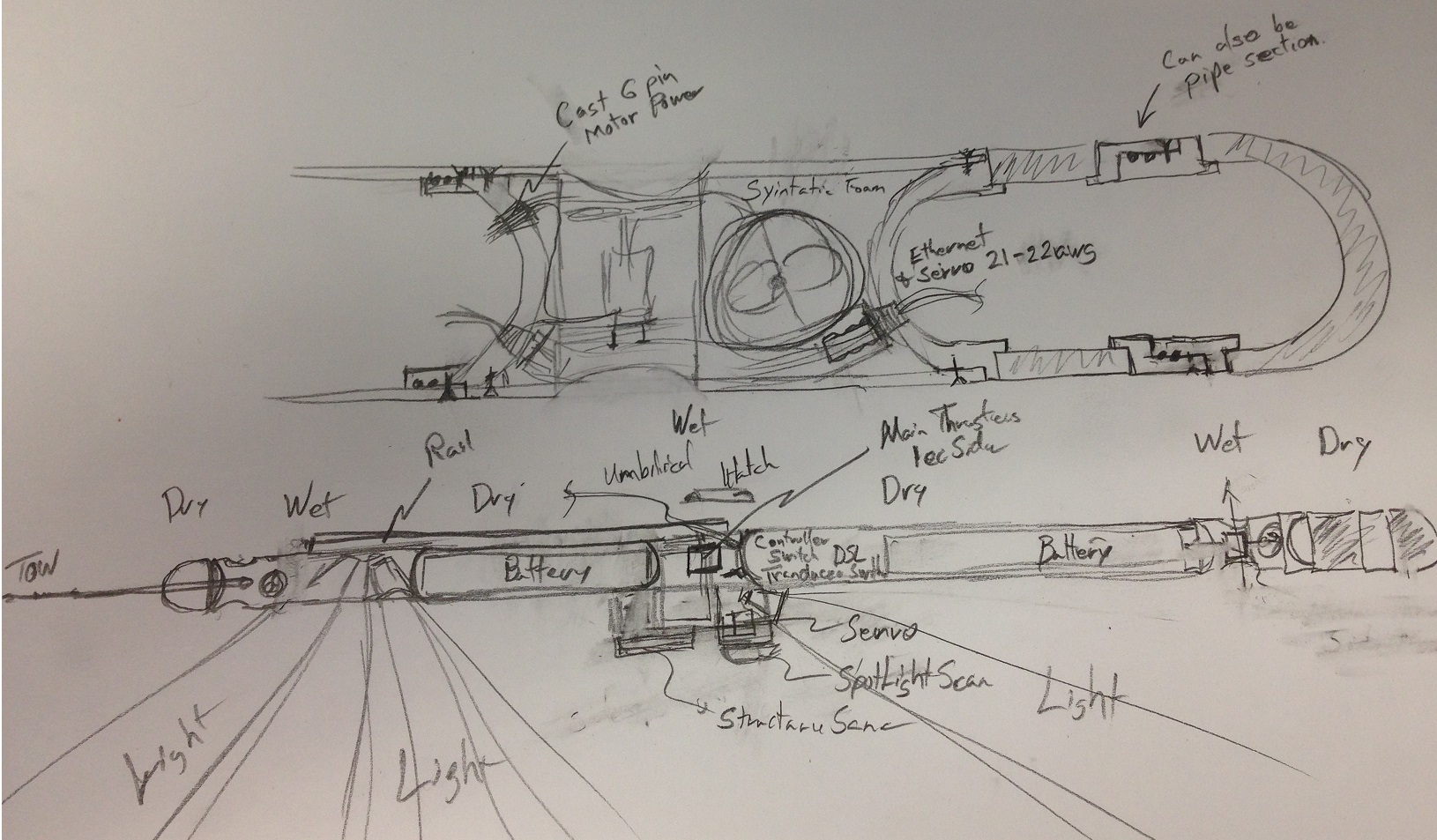
Hull Design 2
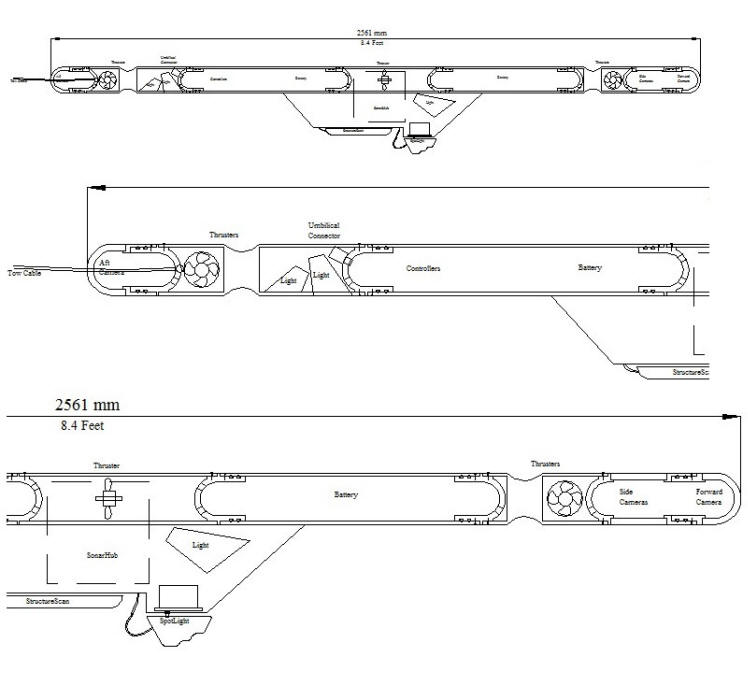
Hull Design 3
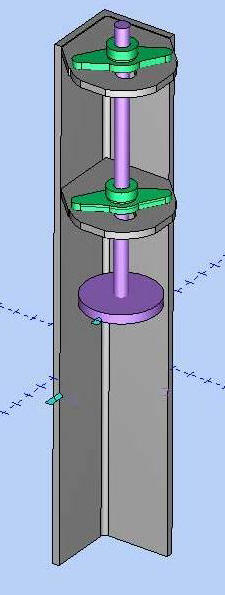
Dave Allen’s Pipe Boring Tool Design
Boring Tool
The hull is devided into seven sections. Four of the sections are 1 atm, dry sections for batteries, cameras, and communication and control equipment. The other three house the thrusters, lights, and sonar equipment which is oversized and so housed in a separate compartment. The 3 1/2″ aluminum pipe used for each section is sealed with o-rings on the couplers that connect each section.
The 3 1/2″ schedule 40 aluminum pipe commonly is out of round by more than 20 thousands. In order to provide a good sealing surface for the o-rings the inside of the pipe needs to be machined round and for a close fit to the coupler. These sections are too long for the average metal lathe so we built a line boring tool to machine the ends of the pipes. Using larger 3/16″ o-rings allows for more errors due to distortion and poor surface finish. These o-rings can easily withstand the high pressure provided there is less than 10 thousands of an inch between the two parts. The line boring tool, designed by Dave Allen, allows us to cut the inside diameter of the pipe so that it is out of round by less than 5 thousands. It uses a piece of angle iron to which the pipe is clamped. Two pillow blocks support a shaft with a cutter head treaded onto one. A hand drill is used to rotate the cutter.
The ROV stuffed with controllers, wires and batteries took it’s first plunge as an assembled unit. At 28 lbs, 12.7 kg negative buoyancy without the wing and 17.6 lbs, 8 kg with the wing; It was heavier than I expected, but then it is stuffed to the gills with batteries and wiring harness. So it is not that surprising. The plan had been to lighten the battery load and add syntactic foam but syntactic foam is expensive, and I like keeping the battery power, so the obvious solution is to simply add additional 3.5″ hull, or hulls above the existing hull. As these would only need to be empty tubes they would be simple to construct and they would greatly add to the rigidity of the lower hull. To add 28 pounds of lift from 3.4″ Sch 40 6061 T6 we need 28/2.16 = 12.96 ft.
Add 3 more feet for end caps, hardware and a trim weight. The total length of the float hull would be 16 ft, 4.88m. The vertical thrusters at each end need to be unobstructed and there is over 8.5 ft, 2.6m between them. A pair of 8.5 ft float hulls would provide more than enough buoyancy even with operating without the dive wing. The mount for the dive wing will need to be redesigned to fit between the two hulls, and a simple plastic bracket designed to fit between the hulls and secure them with hose clamps so that hull penetrations are required.
Notes:
3000 ft operating depth.
3 1/2″ Sch 40 6061-T6 4″ (101.6mm) OD, 3.548″ (90.12mm) ID, 0.452″ (11.48mm) Wall, 3.4lb/ft
Expected Collapse Pressure: 3 1/2″ Sch 40 is 2996 psi / .433 lb/ft = 6,919 ft
Displacement: 5.56 lbs/ft Weight: 3.4 lb/ft Buoyancy: 2.16 lb/ft
4″ Sch 40 6061-T6 4.5″ OD, 4.026″ ID, .237″ Wall, 3.733lb/ft
Expected Collapse Pressure: 4″ Sch 40 is 2694 psi / .433 lb/ft = 6,221 ft
Displacement: d=4.5 r=2.25 area=pi*(2.25^2)=15.9 sq.in. *12=190.85 cu.in * .037 lb/sq.in. seawater =7.06 lb/ft
Displacement: 7.06 lb/ft -Weight: 4 lb/ft = 3.06 lb/ft buoyancy.
Interstate Steel & Metals (918) 585-3844 3 1/2″ Sch 40 6061-T6 4″ OD $175
The Trident Company, 3 1/2″ Sch 40 6061-T6 4″ OD $250
Hull Coupler Designs
Steinn Hrutur came up with a number of possibilities for coupling sections of 3 1/2″ Sch 40 pipe together.
Steinn also pointed out a design problem with regard to using an o-ring as a face seal at the end of a pipe. The problem is that the o-ring compresses leaving less than 1/2 of the wall of the pipe to support the loads applied from both ends, which is not sufficient for 1700 psi.
Our best design at this point is a simple coupler that slips into the pipe using a pair of o-rings, and which is held together with a pair of threaded rods that pass down the top and bottom of the ROV.
O-Ring Seals
3 1/2″ Sch 40 6061-T6 OD: 4″ (101.6mm) OD, ID: 3.548″ (90.12mm) , Wakk: 0.452″ (11.48mm), 3.4lb/ft
ID after machining off about .022: Bore ID: 3.570
Trelleborg Online O-Ring Calculator
Butyl or Silicone
When used as a static seal, the maximum recommended
squeeze for most elastomers is 30%.
Gland fill should be between between 60%
to 85%, 75% is optimum.
In large male gland assemblies, it may be desirable to use an O-ring one size smaller than indicated in the design chart. The design stretch is so small in these large sizes, that the O-ring tends to sag out of the groove before it is assembled. Using the next smaller size simplifies assembly, but requires a reduced gland depth to attain the proper squeeze.
O-ring seal when the groove is cut into a flat surface and when the pressure is inward, the groove inside diameter is primary. This design technique minimizes movement of the O-ring in the groove due to pressure.
The ID of aluminum pipe is not all that accurate, so will the internal coupler can be machined round within a couple of thousands of an inch, it will fit inside a pipe that may vary. A typical Sch40 pipe the ID is 3.548 (“A” in the table above) plus or minus about .04″. So if and interior coupler was turned to and 3.505″ OD (“C” in the table above), it should clear the pipe by at least .003″ and as much as .043″. Will that work without machining the ID of the pipe?
Through Hull Connectors
SeaCon connectors IL-6-FS (Female Side) $41.20ea and the BH-6-MP (Male Side) $61.80, the locking collars are $7 each. seaconworldwide.com
Lowrance SonarHub Housing
Unfortunately the circuit board for the Lowrance SonarHub is one single board just under 7 x 7 inches, so the board must be adapted for the 3 1/2″ ID pipe hull, or the hull must be adapted for the board. Options include cutting the board in half and soldering wire connections between the two sides. Making a 1 ATM housing to protect the boards components from the pressure sounds easy until we do the math. 7 x 7 inches = 49 square inches at 1700 psi = 83,300 pounds. Over 41 tons of force.
1) Spherical Hull
A sphere would be the best shape but it would adversely impact the drag of the hull. Spheres are also require complicated machining processes.
2) Elliptical Hull Supported by Titanium Pins
Some circuit boards have locations on them that are free of IC’s and conductors. These locations could be drilled and have titanium pins that pass through in order to brace one side of a slightly elliptical hull against the other. Grade 5 titanium can has a 138,000 psi of tensile strength. 138000 * (pi*(((1/8)/2)^2)) = 1,693 pounds. The inside of the aluminum hull would need to be machined to within 1/4″ of the surface of the circuit board in order to support flexing of the pins. The titanium pins themselves would be 1/4″ rods turned down to 1/8 as necessary to pass through the 3/32″ circuit board. This would require a complicated machining process.
3) Syntactic Foam Potting
This process ranges from the very simple to the much more complex depending. In it’s simplest form the boar is simply completely coated with mg Chemicals, 832TC-450ML thermally conductive potting resin and placed in a flooded housing make of syntactic form or any other material. The relative thin layer of epoxy and it’s thermally conductive properties avoid issues with overheating and the approach has been proven to work with marine animal tags at 2000 m according to Matthew Valancy with Desert Star. It is also necessary to remove any oils from the conductor pins with Acetone or and an ultrasonic cleaner before potting. The potting can be as thin as 1/16″.
A more complicated approach that may increase the reliability would include multiple coats. The compression strength of a typical FR-4 fiberglass circuit board is 60,200 psi – flatwise. Resin encapsulating 5 micron, 0.0002 inch glass spheres is commonly used as flotation for deep sea vehicles. It has an good compression strength of 8000 psi, but low tensile strength. It also has a much lower shrinkage than typical epoxy. IC’s and capacitors on the circuit board would first be coated with a thin layer of air entrained silicon to form a compressible buffer around these components. The board would then be think coating of “mg Chemicals” 832TC-450ML thermally conductive potting resin which uses aluminum oxide for thermal conduction (.682 W/mK) but which is not electrically conductive. The next layer would be epoxy infused with copper powder to produce a layer with an approximate heat conduction ability of 30 W/mK. This 6 to 10 mm layer will also bond 14 awg solid copper wire to the board in order to conduct heat away from the board. The end of the wires would pass off the edges of the board and be bent to form bands of copper down the sides of the board. Once the copper layer is cured the exposed copper wires would be embedded in salt dough which can be dissolved in water after the final layer of casting is cured. The final outer shell will be cast HYTAC-C syntactic foam poured into a mold of the desired fin shape from 1 to 3 inches thick. After curing the salt dough would be dissolved to form water channels over the exposed copper. This method would also not be reusable should the board fail and need to be replaced but a second board could be potted so replacement is available. This process also simplifies the cable connections as each conductor can by isolated and stripped to bare copper where it passes through the innermost thermally conductive potting layer to form a watertight seal to the conductor. The fin could be installed through a flooded section of the hull to limit it’s cross-section. It could also form a mounting structure for the transducers or a dive plane should the ROV be used without the assistance of a forward towed array that controls depressing the unit when under tow.
4) Aluminum Casting
A circuit board could be covered temporarily with plastic wrap and a female mold of each side of the board made of castable silicone. The silicone negative would then be used to make a wax positive of the board for investment casting in aluminum. The aluminum casting would then be machined for an o-ring to seal between the two halves and have glands fitted for the various connectors. This process like the syntactic form potting described above would rely on the compression strength of the circuit board that is free of components, but would avoid overheating the components. The compression strength of a typical FR-4 fiberglass circuit board is 60,200 psi – flatwise.
5) Aluminum Shell with External Frames and Syntactic Foam Frame Webbing
A two part elliptical aluminum hull with clearance for the board would be machined from one inche solid stock leaving a minimum of 1/2 inch thick material in the hull. External frames of 1/2 thick by 1 inch tall aluminum plate would be welded onto the exterior of the hull and 3/8 inch lightening holes would be drilled through the frames. An temporary exterior skin would be attached to the top of the frames and the cavity between the skin and the hull filled with syntactic foam. The lightening holes would allow the syntactic foam to attach to the frames. The compression strength of the foam would act like a webbing for the frames in order to resist buckling.
6) Cut the Circuit Board and Soldier it Back Together
It an interesting idea, but a long shot, but it is possible to cut circuit boards apart and join the pieces with flexible wire or ribbon conductors. However this board processes very low power analog signals from the transducer which complicates this process.
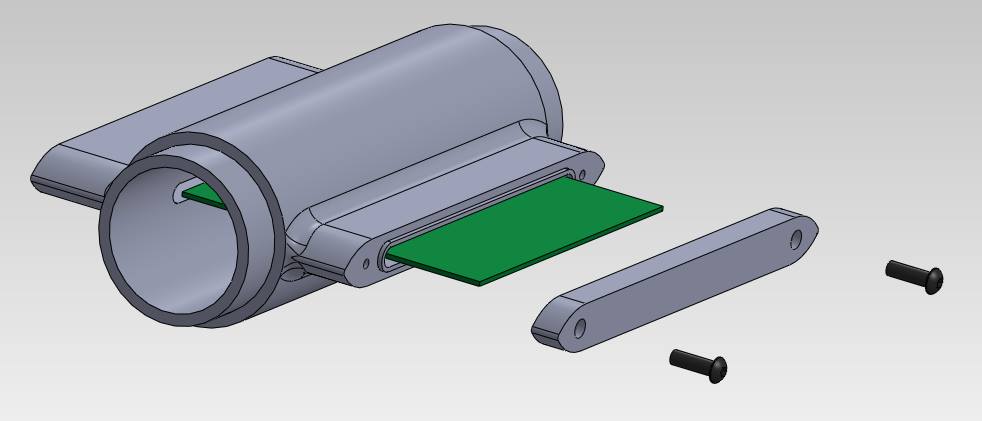
Cast Circuit Board Hull Housing -by Drew Smith
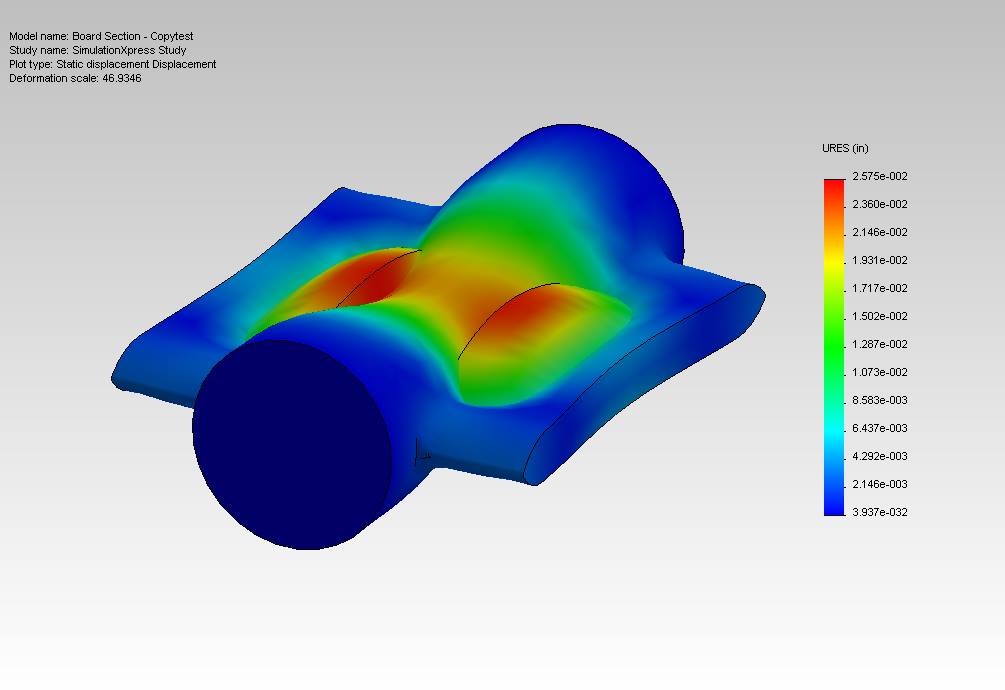
Rough simulation, viewing the ends as solid
4″ OD x 2″ thick 356 aluminum. Greatest
deflection is .025″
7) Cast Circuit Board Hull Housing
A complete section of the hull with winglets to house the circuit board can be cast from aluminum. The winglets would protrude from both sides of the 4″ OD pipe section so as to minimize their surface area. One of the wing tips would be capped with a bolt retained hatch.
8) Oil Compensated Housing
A very simple solution is to build a light weight housing for the board, and flood it with mineral oil. A flexible hose or diaphragm is connected to the housing that allows the oil to be compressed to match the external pressure.
Woods Hole use mineral oil in all PBOF (Pressure balanced/oil filled) systems. Thrusters have NNL690-G Oil Fortifier added for lubrication. $74/gallon It’s normally used in gear boxes and transmissions. http://poweruplube.com/nnl690-g-oil-fortifier
Syntactic Foam:
Engineered Syntactic Systems
Thomas J Murray
CMT Materials, Inc
www.esyntactic.com
www.cmtmaterials.com
+1 (508) 226 3901
Castable-Syntactic
HYTAC-C-Casting-Curing
HYTAC-C Castable Syntatic Foam | |
Density (ρ) | 44 lb/ft3 [705 kg/m3] |
Thermal Conductivity (k) | 0.16 BTU/hr-ft-°F [0.18 W/m°K] |
Coefficient of Thermal Expansion(CTE) | 17 x 10-6 in/in° F [67.7 x 10-6m/m/°C] |
Compressive Strength | 8,000 psi [55 Mpa] |